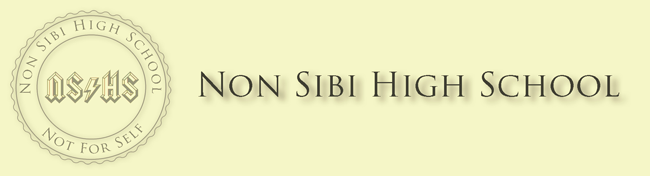
Chapter 9: Chemical Bonding and Nomenclature
Section 9-1: Relationship Between Groups and Number of Valence Electrons
Section 9-2: Electronegativity
Section 9-3: Ionic Bonding and Ionic Nomenclature
Section 9-4: Molecular Compounds, Covalent Bonding, and Lewis Structures
Section 9-5: Bond Length and Resonance
Section 9-6: Nomenclature of Binary Molecular Compounds
Section 9-7: Introduction to Organic Nomenclature
Section 9-8: Names of Common Acids
Chapter 9 Practice Exercises and Review Quizzes
Section 9-1: Relationship Between Groups and Number
of Valence Electrons
For Groups 1, 2, and 13 through 18
(except helium), all elements in a group will have exactly the same number of
valence s and p electrons.
Therefore, we can quickly determine the total number of valence
electrons using the following chart:
Group
|
Valence Electron Configuration
|
Total Valence Electrons
|
1
|
ns1
|
1
|
2
|
ns2
|
2
|
13
|
ns2 np1
|
3
|
14
|
ns2 np2
|
4
|
15
|
ns2 np3
|
5
|
16
|
ns2 np4
|
6
|
17
|
ns2 np5
|
7
|
18
|
ns2 np6
|
8
|
Note that any d or f electrons that
an element may possess will have a lower value of n than the valence electrons
and, therefore, should not be included in the valence electron configuration or
the total number of valence electrons.
Although most transition metals in groups 3 through 12 will have a
valence electron configuration of ns2 and, therefore, a total of 2
valence electrons, there are numerous exceptions.
Section 9-2: Electronegativity
Electronegativity (EN) is
the ability of an atom to attract electrons in a chemical bond. Electronegativity,
which is relative and has no unit, generally increases toward the upper right
corner of the periodic table table, with fluorine
having the highest electronegativity value of all
elements. The following table
shows the electronegativity values of select
elements:
Group 1
|
Group 2
|
Groups 3-12
|
Group 13
|
Group 14
|
Group 15
|
Group 16
|
Group 17
|
Group 18
|
H
2.1
|
|
|
|
|
Li
1.0
|
|
B
2.0
|
C
2.5
|
N
3.0
|
O
3.5
|
F
4.0
|
|
Na
0.9
|
Mg
1.2
|
Al
1.5
|
Si
1.8
|
P
2.1
|
S
2.5
|
Cl
3.0
|
|
K
0.8
|
Ca
1.0
|
|
|
As
2.0
|
Se
2.4
|
Br
2.8
|
Kr
3.0
|
Rb
0.8
|
Sr
1.0
|
|
|
|
Te
2.1
|
I
2.5
|
Xe
2.6
|
Cs
0.8
|
Ba
0.9
|
|
|
|
|
|
|
Fr
0.7
|
|
|
|
|
|
|
|
For purposes of chemical bonding,
the elements in bold print toward
the lower left corner with relatively low electronegativities
will generally be considered metals, while the elements in italics toward the upper right corner with relatively high electronegativities will generally be considered
nonmetals. Note that hydrogen has
a relatively high electronegativity and, therefore,
should be considered a nonmetal.
Section 9-3: Ionic Bonding and Ionic Nomenclature
When a metal with a low electronegativity reacts with a nonmetal with a high electronegativity, the large difference in electronegativity (ΔEN) allows for the metal to transfer
one or more electrons to the nonmetal.
As a result, the metal becomes a positively-charged cation
and the nonmetal becomes a negatively-charged anion. The attraction between the cation
and anion is known as ionic bonding.
In the case of a Group 1 alkali
metal such as lithium, a Group 2 alkaline earth metal such as calcium, or
aluminum metal, the metal will lose all its valence electrons and become a cation with a noble gas electron configuration:
Li: [He] 2s1 loses 1 valence electron
to become Li+ : [He]
Ca: [Ar] 4s2 loses 2 valence
electrons to become Ca2+ :
[Ar]
Al: [Ne] 3s2 3p1
loses 3 valence electrons to become Al3+ : [Ne]
Nonmetals in Group 15 such
phosphorus, Group 16 such as oxygen, and Group 17 such as bromine will gain
enough electrons to have an electron configuration ending in np6
that is equivalent to the electron configuration of the noble gas nearest in
atomic number to the nonmetal:
P: [Ne] 3s2 3p3
gains 3 electrons to become P3- :
[Ne] 3s2 3p6 or [Ar]
O: [He] 2s2 2p4
gains 2 electrons to become O2- :
[He] 2s2 2p6 or [Ne]
Br: [Ar] 4s2 3d10 4p5
gains 1 electron to become Br- : [Ar] 4s2 3d10 4p6
or [Kr]
From the discussion above, we can
conclude the following about monatomic ions:
Ion
|
Charge
|
Group 1
alkali metal cation
|
1+
|
Group 2
alkaline earth metal cation
|
2+
|
aluminum metal cation
|
3+
|
Group 15
nonmetal anion
|
3-
|
Group 16
nonmetal anion
|
2-
|
Group 17
nonmetal anion
|
1-
|
The nomenclature or naming of an
ionic compound formed by combining one type of metal cation
from the table above and one type of nonmetal anion from the table above will
follow the format:
(name of metal) +
(name of nonmetal with "ide" ending)
To determine the chemical formula
of an ionic compound, we must choose the correct number of cations
and the correct number of anions to ensure that the total positive charge of
the cations equals the total negative charge of the
anions, and we will typically write the cation
first in the formula. When
the magnitude of the positive charge of the cation
equals the magnitude of the negative charge of the anion, the formula will
contain one cation and one anion. Note, however, that the final written formula does not
actually show charges:
sodium chloride = (combine Na+ with Cl-) = NaCl
magnesium sulfide = (combine Mg2+ with S2-)
= MgS
aluminum nitride = (combine Al3+ with N3-)
= AlN
When the magnitude of the positive
charge of the cation does not equal the magnitude of
the negative charge of the anion, we must use subscripts in the formula to
adjust the number of ions in order to equalize the total positive and negative
charges. For example:
potassium oxide = (combine 2 K+ with 1 O2-)
= K2O
aluminum fluoride = (combine 1 Al3+ with 3 F-)
= AlF3
calcium phosphide = (combine 3 Ca2+
with 2 P3-) = Ca3P2
Sample Exercise 9A:
Write the chemical formula for each
ionic compound:
(a) barium
chloride
(b) aluminum
sulfide
(c) lithium
nitride
Solution:
(a) (combine
1 Ba2+ with 2 Cl-) = BaCl2
(b) (combine
2 Al3+ with 3 S2-) = Al2S3
(c) (combine
3 Li+ with 1 N3-) = Li3N
Most other metals can achieve more
than one positive charge. To
distinguish among the possible cations for these
other metals, the name of the metal will be followed by the magnitude of the
positive charge in Roman numerals enclosed by parentheses. For example, iron(III) = Fe3+
and tin(IV) = Sn4+.
Groups of bonded atoms that possess
an overall charge are known as polyatomic
ions. Here is a list of common
polyatomic ions:
1+
ammonium = NH4+
1-
acetate = CH3COO- or C2H3O2-
bicarbonate or hydrogen carbonate = HCO3-
hydroxide = OH-
nitrate = NO3-
2-
carbonate = CO32-
sulfate = SO42-
3-
phosphate = PO43-
When writing chemical formulas for
ionic compounds containing polyatomic ions and a subscript greater than 1 is
required after the formula of a polyatomic ion, the formula of the polyatomic
ion should be enclosed in parentheses.
For example:
aluminum acetate = (combine 1 Al3+ with 3 CH3COO-)
= Al(CH3COO)3
nickel(II) phosphate = (combine 3 Ni2+ with 2 PO43-)
= Ni3(PO4)2
ammonium sulfate = (combine 2 NH4+ with
1 SO42-) = (NH4)2SO4
Sample Exercise 9B:
Write the chemical formula for each
ionic compound:
(a) cobalt(III)
carbonate
(b) strontium
hydroxide
(c) ammonium
nitride
Solution:
(a) (combine
2 Co3+ with 3 CO32-) = Co2(CO3)3
(b) (combine
1 Sr2+ with 2 OH-) = Sr(OH)2
(c) (combine
3 NH4+ with N3-) = (NH4)3N
If we are
given the chemical formula of an ionic compound and need to write the name, we
will use the format:
(ammonium or name of metal)
+
(name of polyatomic anion or name of nonmetal with "ide"
ending)
Remember that in all but a few
cases the name of the metal should include the magnitude of the charge in Roman
numerals enclosed by parentheses, except if the metal is in Group 1, in Group
2, or aluminum.
Sample Exercise 9C:
Write the name of each compound:
(a) Mn(HCO3)2
(b) Al(NO3)3
(c) (NH4)2S
Solution:
(a) Since the bicarbonate or
hydrogen carbonate ion is known to have a 1- charge and there are two such ions
in the formula, we can deduce that the charge on the metal must be 2+. Therefore, the name of the compound is
manganese(II) bicarbonate or manganese(II) hydrogen carbonate.
(b) We do not need to specify the
charge of aluminum in Roman numerals enclosed by parentheses, so the name of
the compound is aluminum nitrate.
(c) The anion S2- is
simply a monatomic nonmetal anion, not to be confused with the polyatomic anion
sulfate, so the name of the compound is ammonium sulfide.
On a final note, the term
"molecule" should not be used to describe an individual unit of an ionic
compound such as NaCl. The more appropriate term "formula unit" should be used
instead.
Section 9-4: Molecular Compounds, Covalent Bonding,
and Lewis Structures
Molecular compounds are composed of
nonmetals. When two nonmetals are
bonded, since each has a relatively high electronegativity,
the small difference in electronegativity precludes
the transfer of electrons that was possible in ionic compounds. Instead, the two nonmetals will share
one or more pairs of valence electrons in a covalent bond.
A Lewis structure depicts each shared pair of valence electrons in a
molecule using a straight line between the bonded atoms. In addition, the unshared pairs of
valence electrons in a molecule, known as a lone pairs, are depicted in a Lewis structure using one pair of
dots per lone pair. To draw a
Lewis structure, we will follow the steps below as demonstrated for the
molecule Br2:
Lewis structure Step 1:
Determine the sum of the valence electrons for each atom in the molecule
or ion. For cations,
subtract the magnitude of the positive charge from the total. For anions, add the magnitude of the
negative charge to the total. The
final Lewis structure must have this total number of electrons.
Br is in Group 17, so each Br atom
has 7 valence electrons.
Therefore, the Lewis structure for Br2 must have a total of 2 x 7 = 14
electrons.
Lewis structure Step 2:
Connect each bonded atom by a single covalent bond.
Draw a straight line between the
atoms to represent a shared pair of electrons:
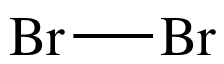
Lewis structure Step 3:
Place lone pairs in separate locations adjacent to each outer atom, but
never between atoms, until each outer atom has an octet of 8 valence electrons
adjacent to it, thus achieving the "Octet Guideline" of having the same number
of valence electrons as all noble gases, except helium.
Place 3 pairs of dots in separate
locations adjacent to each Br atom, but not between the Br atoms, to represent
3 lone pairs of electrons on each Br:
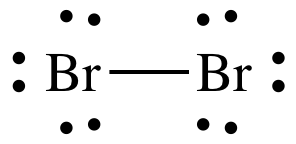
The Lewis structure of Br2 is
now complete with the correct total of 14 electrons and each Br atom having an
octet of 8 adjacent electrons, 2 shared electrons in the covalent bond + 6
electrons in the 3 lone pairs.
Two bonded nonmetals with similar electronegativities are said to be joined by a nonpolar covalent bond where the electrons in
the bond are essentially attracted equally to each atom and, therefore, spend
roughly equal amounts of time near each of the two atoms. When the two bonded nonmetals have a
very small electronegativity difference of 0.4 or
less, such as that between hydrogen (EN = 2.1) and carbon (EN = 2.5), we will
generally consider the bond between the nonmetals to be a nonpolar
covalent bond. On the other hand,
if the electronegativity difference between the
bonded nonmetals is 0.5 or greater, we will generally consider the bond between
the nonmetals to be a polar covalent
bond where the electrons in the bond are attracted unequally to each atom
and, therefore, spend more time closer to one atom and farther away from the
other atom.
Although the electronegativity
of hydrogen is high enough for hydrogen to be considered a nonmetal, there is a
large enough difference in electronegativity between
the hydrogen and oxygen atoms in water (EN for O = 3.5, so ΔEN between H and O
= 1.4) for the bonds in water to be considered polar covalent bonds. To draw the Lewis structure of H2O,
we begin by performing the first two steps above:
Step 1: Each H has 1 valence electron. O is in Group 16, so the O atom has 6 valence
electrons. Therefore, the Lewis
structure of water H2O must have a total of (2 x 1) + 6 = 8
electrons.
Step 2: When a single atom of one element is bonded to two or more
atoms of a second element, we will generally place the single atom in the
center of the Lewis structure and spread the other atoms around the
center. As such, we will place the
oxygen atom in the center and connect to each hydrogen atom with a single
covalent bond:
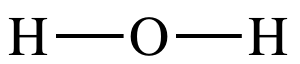
At this point, we will skip step 3
and introduce an important exception to the "Octet Guideline" as well as the
fourth step in the process of drawing Lewis structures:
"Octet Guideline" Exception 1: Since the noble gas nearest in atomic number to hydrogen is
helium, which has an electron configuration of 1s2 and 2 valence
electrons, H will always have an
incomplete octet of 2 valence electrons in Lewis structures. As such, H must always be an outer atom
joined to only one other atom by a single covalent bond.
Lewis structure Step 4A:
Place lone pairs in separate locations adjacent to the center atom, but
never between atoms, until the correct total number of electrons has been
reached in the Lewis structure.
Step 4A: Place two lone pairs adjacent to the center O atom, but not
between atoms, to reach the correct total of 8 electrons:

The Lewis structure of water is now
complete with the correct total of 8 electrons and the O atom having an octet
of 8 adjacent electrons, 4 shared electrons in the 2 covalent bonds + 4
electrons in the 2 lone pairs.
Below are two more notable
exceptions to the "Octet Guideline":
"Octet Guideline" Exception 2:
Lewis structures with boron having an incomplete octet of 6 electrons
are preferable, although boron may also have an octet in some cases.
To draw the Lewis structure of BI3,
we follow steps 1 through 3 above:
Step 1: B is in Group 13, so B has 3 valence electrons. I is in Group 17, so each I has 7
valence electrons. Therefore, the
Lewis structure must have a total of 3 + (3 x 7) = 24 electrons.
Step 2: Place the single B atom in the center and spread the three I
atoms around the B, then connect the B to each of the three I atoms by single
covalent bonds:
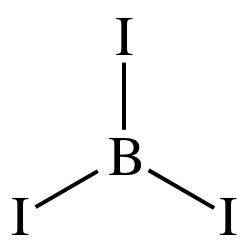
Step 3: Place lone pairs on the I atoms until each has an octet:

The Lewis structure now has the
correct total of 24 electrons, but we did not perform step 4A because there
were no more electrons to place on the center B atom. Since it is preferable to leave B with an incomplete octet
of 6 electrons, the Lewis structure is now complete.
"Octet Guideline"
Exception 3: The center atom in a
Lewis structure can exceed 8 electrons and have an "expanded octet" only if the
center atom is NOT in the second row of the periodic table.
To draw the Lewis structure of the
PCl4- anion, we follow steps 1-4A above:
Step 1: P is in Group 15, so P has 5 valence electrons. Cl is in
group 17, so each Cl has 7 valence electrons. We add one extra electron because the
charge on the anion is 1-, so the Lewis structure must have a total of 5 + (4 x
7) + 1 = 34 electrons.
Step 2: Place the single P atom in the center and spread the four Cl atoms around the P, then connect the P to each of the
four Cl atoms by single covalent bonds:
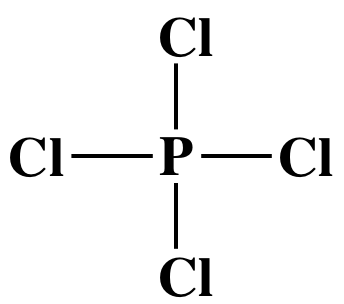
Step 3: Place lone pairs on the Cl atoms
until each has an octet:
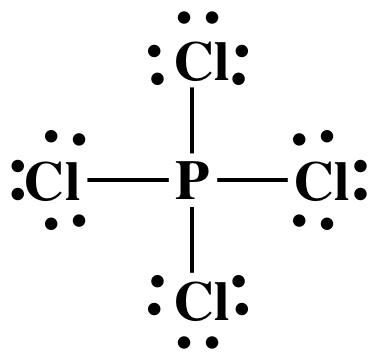
Step 4A: Place one lone pair on the center P atom to reach the
correct total of 34 electrons:

The Lewis structure is now complete
with the center atom P having an "expanded octet" of 10 electrons, which is
acceptable because P is not in the second row of the periodic table. Note that Lewis structures of ions are
typically enclosed in brackets with the charge shown outside the bracket as a
superscript.
Sample Exercise 9D:
In some cases, noble gases can form
compounds. Draw the Lewis
structure for KrF2.
Solution:
8 + (2 x 7)
= 22 electrons
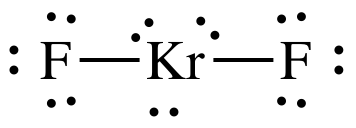
Kr has an "expanded octet" of 10
electrons, which is acceptable because Kr is not in the second row of the
periodic table.
In addition to single covalent
bonds with one pair of shared electrons between the atoms, it is also possible
for two nonmetals to covalently bond with two or three shared pairs of
electrons between the atoms. For
example, O2 has a double bond depicted by two straight lines between
the oxygen atoms in the Lewis structure, and HCN contains a triple bond
depicted by three straight lines between the carbon and nitrogen atoms in the
Lewis structure.
To draw the Lewis structure of O2,
we can treat one of the O atoms as a center atom and the other as an outer atom
as we follow steps 1 through 4A above:
Step 1: 2 x 6 = 12 electrons
Step 2: Connect O atoms by single covalent bond:
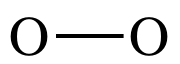
Step 3: Treating the oxygen atom on the right as an outer atom,
place lone pairs to give the O on the right an octet:
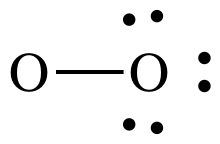
Step 4A: Treating the oxygen atom on the left as the center atom,
place lone pairs on the O to the left until the correct total of 12 electrons
is reached:
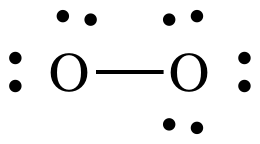
At this point, the O on the left
has an incomplete octet of only 6 electrons, but we have no more electrons to
add. As such, we must introduce
another step in the process of drawing Lewis structures:
Lewis structure Step 4B:
Move one or more lone pairs from outer atoms to create multiple bonds
until the center atom has an octet.
Move any one of the three lone
pairs on the right-hand O between the atoms to create a double bond:
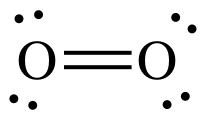
The Lewis structure is now complete
with each O having an octet, 4 shared electrons in the double bond + 4
electrons in the 2 lone pairs.
To draw the Lewis structure of BI3,
it was not necessary to use Step 4A or 4B. To draw the Lewis structure of PCl4-,
it was necessary to use Step 4A but not Step 4B. To draw the Lewis structure of O2, we used both
Step 4A and Step 4B. To draw the
Lewis structure of HCN, we will need to skip Step 4A due to lack of electrons
and only use Step 4B to achieve an octet on the center C atom:
Step 1: 1 + 4 + 5 = 10 electrons
Step 2: Connect center C to H and N by single covalent bonds:
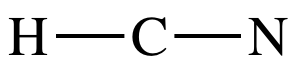
Step 3: Since H must have an incomplete octet of 2 electrons, place
lone pairs only on N to give N an octet:
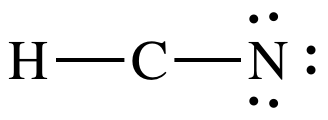
At this point, C only has 4
electrons, but we must skip Step 4A because there are already 10 total
electrons and there are no more electrons to add. As such, we must proceed to Step 4B.
Step 4B: Move two lone pairs from the N to create a triple bond
between the C and N atoms:
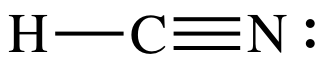
The
Lewis structure is now complete with the center C having an octet, 6 shared
electrons in the triple bond + 2 shared electrons in the single bond.
Section 9-5: Bond Length and Resonance
Bond length is the distance between the nuclei of two covalently
bonded atoms. As the number of
shared electron pairs increases, the nuclei of the two atoms will be drawn
closer together and the bond length will decrease:
number of bonds ↑ = bond length ↓
|
Sample Exercise 9E:
Draw Lewis structures for N2
and N2H2.
Which molecule will have the shorter nitrogen-to-nitrogen bond length? Explain briefly.
Solution:
Step 1: N2 = 2 x 5 = 10 electrons
Steps 2 and 3: Connect N atoms by single covalent bond
and, treating the N on the right as an outer atom, place lone pairs to give the
N on the right an octet:
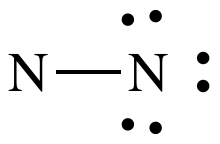
Step 4A: Treating the N on the left as the center atom, place one
lone pair on the left-hand N to reach 10 total electrons:
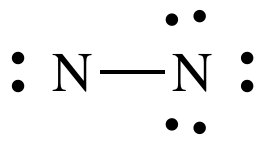
Step 4B: Move two lone pairs from the N on the right to create a
triple bond between the N atoms:
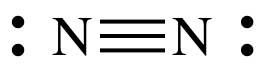
The
Lewis structure for N2 is now complete with each N having an octet,
6 shared electrons in the triple bond + 2 electrons in the lone pair.
Step
1: N2H2 = (2
x 5) + (2 x 1) = 12 electrons
Step
2: Each H must be an outer atom,
so connect the two N atoms by a single covalent bond and then connect one H to
each N by a single covalent bond:

Step
3: Treating the N on the right as
an outer atom, place lone pairs to give the N on the right an octet:
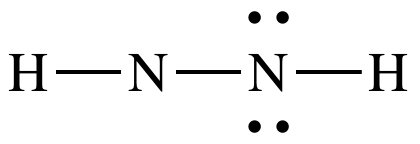
Step
4A: Treating the N on the left as
the center atom, place one lone pair on the left-hand N to reach 12 total
electrons:
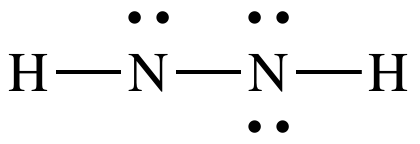
Step
4B: Move one lone pair from the N
on the right to create a double bond between the N atoms:

The
Lewis structure for N2H2 is now complete with each N
having an octet, 4 shared electrons in the double bond + 2 shared electrons in
the single bond + 2 electrons in the lone pair.
Since
the number of bonds in N2's triple bond is greater than the number
of bonds in N2H2's double bond, N2 will have
the shorter nitrogen-to-nitrogen bond length.
We
will now demonstrate the concept of resonance by drawing the Lewis structure of
ozone, O3:
Step
1: 3 x 6 = 18 electrons
Steps
2 and 3:
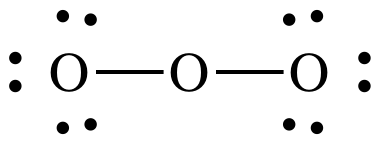
Step
4A:
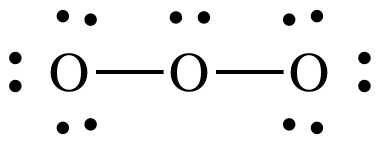
At
this point, we have the correct total of 18 electrons, but the center O has an
incomplete octet of only 6 electrons.
For Step 4B, we can move a lone pair from either outer O atom to create
a double bond and give the center O atom an octet. When double or triple bonds can be formed from different
outer atoms, the resulting Lewis structures are known as resonance structures and should be connected by a double-headed
arrow:

If
either the Lewis structure on the left or the Lewis structure on the right was
alone and represented the true structure of ozone, then one of the O-to-O bond
lengths would be shorter than the other due to the different number of bonds
between the O atoms. However,
experimental evidence suggests that both O-to-O bond lengths are exactly equal
in length and shorter than an O-to-O single bond but longer than an O-to-O
double bond. As such, the true
structure of ozone cannot be either one of the Lewis structures above and
instead must be an equal hybrid of the two resonance structures shown above.
Sample
Exercise 9F:
Draw
all the resonance structures for the carbonate ion.
Solution:
Step 1: CO32- = 4 + (3 x 6) + 2 = 24 electrons
Steps
2 and 3:
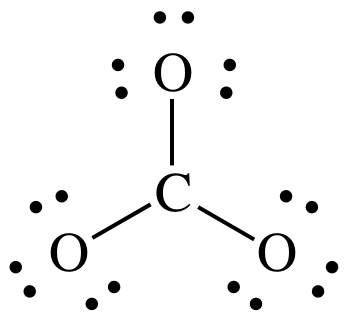
At
this point, we have the correct total of 24 electrons, but the center C atom
has an incomplete octet of 6 electrons.
We must skip Step 4A because there are no more electrons to add to the
center C.
Step
4B:

Experimental
evidence suggests that all three C-to-O bond lengths are exactly equal in
length and shorter than a C-to-O single bond but longer than a C-to-O double
bond. As such, the true structure
of the carbonate ion cannot be any one of the three Lewis structures above and
instead must be an equal hybrid of all three resonance structures shown above.
Section
9-6: Nomenclature of Binary
Molecular Compounds
The
common nomenclature of binary molecular compounds composed of two different
nonmetals utilizes one of the following prefixes to specify the number of atoms
of the first element in the formula and a second prefix from below to specify
the number of atoms of the second element in the formula:
Prefix
|
Number of
Atoms
|
mono
|
1
|
di
|
2
|
tri
|
3
|
tetra
|
4
|
penta
|
5
|
hexa
|
6
|
Note
the following:
1.
Whereas the name of the first element in the formula is unchanged, the name of
the second element in the formula will have the "ide" ending. For example, N2O3
= dinitrogen trioxide.
2. The prefix "mono" is typically omitted
if there is only one atom of the first element in the formula. For example, BrI5 = bromine
pentaiodide.
3. However, the prefix "mono" is used if
there is only one atom of the second element in the formula, but the prefix is
typically truncated to "mon" when followed by "oxide." For example, CO = carbon monoxide.
4.
The prefixes "tetra," "penta," and "hexa" are typically truncated by dropping
the "a" when followed by "oxide."
For example, P4O6 = tetraphosphorus hexoxide.
Section
9-7: Introduction to Organic
Nomenclature
Organic
compounds contain carbon. For some
categories of organic compounds, the following prefixes are used to specify the
number of carbon atoms in the chemical formula:
Prefix
|
Number of
Carbon Atoms
|
meth
|
1
|
eth
|
2
|
prop
|
3
|
but
|
4
|
pent
|
5
|
hex
|
6
|
hept
|
7
|
oct
|
8
|
Alkanes are organic compounds composed of single-bonded carbon atoms surrounded
by hydrogen atoms. The common
nomenclature of alkanes begins with one of the prefixes above to specify the
number of carbon atoms and then the suffix "ane." To obtain the condensed chemical formula of an alkane,
substitute the number of carbons for n in the formula CnH2n+2. For example, the fuel propane has n = 3
and, thus, a formula of C3H8.
Alcohols are organic compounds composed of single-bonded carbon atoms surrounded
by hydrogen atoms, but one of the carbons is single-bonded to an OH group. The common nomenclature of alcohols
begins with one of the prefixes above to specify the number of carbon atoms and
then the suffix "anol" or "yl alcohol."
To obtain the partially-condensed chemical formula of an alcohol,
substitute the number of carbons for n in the formula CnH2n+1OH. For example, ethanol or ethyl alcohol
has n = 2 and, thus, a formula of C2H5OH.
Section
9-8: Names of Common Acids
In
different aqueous acids, hydrogen ions (H+) are present in all of
the acid solutions, but different anions will be present in each of the
different acid solutions. Here are
the names of some common acids:
HCl = hydrochloric acid
HNO3 = nitric acid
H2SO4 = sulfuric acid
CH3COOH or HCH3COO or HC2H3O2
= acetic acid
Chapter 9 Practice Exercises and Review Quizzes:
9-1) Write
the chemical formula for each ionic compound:
(a) ammonium
phosphide
(b) chromium(III)
nitrate
(c) aluminum
carbonate
9-1) (a)
(combine 3 NH4+ with 1 P3-) = (NH4)3P
(b) (combine 1 Cr3+
with 3 NO3-) = Cr(NO3)3
(c) (combine 2 Al3+
with 3 CO32-) = Al2(CO3)3
9-2) Write
the name of each ionic compound:
(a) Ca(CH3COO)2
(b) K2SO4
(c) CuOH
9-2) (a)
Ca2+ = Group 2 cation = no Roman numerals,
so calcium acetate
(b) K+
= Group 1 cation = no Roman numerals, so potassium
sulfate
(c) Since
hydroxide is known to be 1-, the metal ion must be 1+, so
copper(I) hydroxide.
9-3) Draw Lewis structures for each
of the following:
(a) boron trifluoride
(b) IBr4-
9-3) (a)
BF3 = 3 + (3 x 7) = 24 electrons
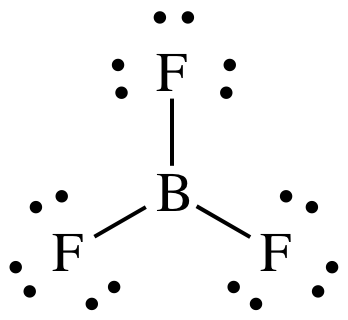
It is preferable for B to have an
incomplete octet of 6 electrons.
(b) 7 + (4 x 7) + 1 = 36 electrons
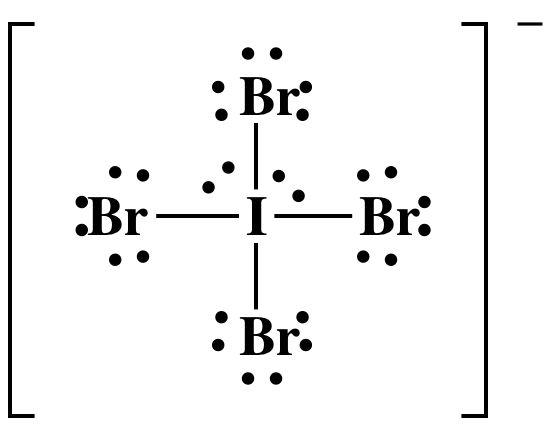
I has an
"expanded octet" of 12 electrons, which is acceptable because I is not in the
second row of the periodic table.
9-4) Draw Lewis structures for C2H2
and C2H4.
Which molecule will have the longer carbon-to-carbon bond length? Explain briefly.
9-4) C2H2 =
(2 x 4) + (2 x 1) = 10 electrons:

C2H4 = (2 x 4) + (4
x 1) = 12 electrons:

Since
the number of bonds in C2H4's double bond is less than
the number of bonds in C2H2's triple bond, C2H4
will have the longer carbon-to-carbon bond length.
9-5) Draw
all the resonance structures for the nitrate ion.
9-5)
NO3- = 5 + (3 x 6) + 1 = 24 electrons
9-6) Write
the chemical formula for each binary molecular compound:
(a) sulfur
hexafluoride
(b) phosphorus
pentachloride
9-7) Write
the name of each binary molecular compound:
(a) N2O
(b) N2O4
9-7) (a) dinitrogen monoxide
(b) dinitrogen tetroxide
9-8) Write
the chemical formula for each organic compound:
(a) methanol
or methyl alcohol
(b) butane
9-9) Write
the name of each organic compound:
(a) C8H18
(b) C5H11OH
9-9) (a)
octane
(b) pentanol or pentyl
alcohol
Click for Review Quiz 1
Click for Review Quiz 1 Answers